Engineered microorganisms
Principles of Genetic Engineering in Microorganisms
The core principle underlying the engineering of microorganisms is the manipulation of their genetic material, primarily DNA. This involves a series of steps:
- Gene Isolation: The desired gene, which encodes the desired trait, is isolated from the source organism. This can be achieved through various techniques, such as polymerase chain reaction (PCR) and restriction enzyme digestion.
- Vector Construction: The isolated gene is inserted into a vector, which acts as a carrier to transport the gene into the host microorganism. Commonly used vectors include plasmids, bacteriophages, and cosmids.
- Transformation: The vector carrying the desired gene is introduced into the host microorganism. This process, known as transformation, can be achieved through various methods, such as electroporation, chemical treatment, and conjugation.
- Selection and Screening: Transformed microorganisms are selected and screened to identify those that have successfully incorporated the desired gene. This is often achieved using antibiotic resistance markers or other selectable markers.
- Expression and Characterization: The engineered microorganism is cultured to express the desired trait. The expression of the new trait is then characterized to ensure that it functions as intended.
Applications of Engineered Microorganisms
The applications of engineered microorganisms are diverse and span a wide range of fields:
- Medicine:
Antibiotic Production: Engineered microorganisms are used to produce a wide range of antibiotics, including penicillin, erythromycin, and tetracycline. Genetic engineering techniques have been used to improve the yield and efficiency of antibiotic production.
Vaccine Development: Engineered microorganisms can be used to produce vaccines against various infectious diseases. For example, the hepatitis B vaccine is produced using yeast cells that have been genetically engineered to express the hepatitis B surface antigen.
Gene Therapy: Engineered microorganisms can be used as vectors to deliver therapeutic genes to target cells in the body. This approach holds promise for the treatment of genetic disorders such as cystic fibrosis and muscular dystrophy.
Cancer Treatment: Engineered microorganisms can be used to target and destroy cancer cells. For example, some bacteria have been engineered to selectively target and kill tumor cells.
Bioremediation: Engineered microorganisms can be used to clean up environmental pollutants, such as oil spills and heavy metals. For example, some bacteria have been engineered to degrade toxic chemicals, such as polychlorinated biphenyls (PCBs).
- Agriculture:
Crop Improvement: Engineered microorganisms can be used to improve crop yields and resistance to pests and diseases. For example, some bacteria have been engineered to produce insecticidal proteins, which can protect crops from insect damage.
Biofertilizers: Engineered microorganisms can be used to improve soil fertility and promote plant growth. For example, some bacteria have been engineered to fix nitrogen from the atmosphere, making it available to plants.
Animal Feed: Engineered microorganisms can be used to improve the nutritional value of animal feed. For example, some bacteria have been engineered to produce enzymes that can break down complex carbohydrates, making them more digestible for animals.
- Industry:
Biofuel Production: Engineered microorganisms can be used to produce biofuels, such as ethanol and biodiesel, from renewable resources. For example, some bacteria have been engineered to convert plant biomass into ethanol.
Bioremediation: Engineered microorganisms can be used to clean up industrial waste and pollution. For example, some bacteria have been engineered to degrade toxic chemicals, such as pesticides and herbicides.
Food Production: Engineered microorganisms are used in the food industry to produce a variety of products, including cheese, yogurt, and bread. Genetic engineering techniques have been used to improve the flavor, texture, and shelf life of these products.
Biopharmaceuticals: Engineered microorganisms are used to produce a wide range of biopharmaceuticals, including insulin, human growth hormone, and interferon. Genetic engineering techniques have been used to improve the yield and purity of these products.
Ethical and Safety Considerations
The development and application of engineered microorganisms raise a number of ethical and safety considerations:
Environmental Impact: The release of engineered microorganisms into the environment could have unintended consequences, such as the spread of antibiotic resistance or the disruption of ecosystems.
Human Health: The use of engineered microorganisms in medicine and agriculture could pose risks to human health, such as allergic reactions or the development of new diseases.
Social and Economic Implications: The widespread use of engineered microorganisms could have significant social and economic implications, such as the displacement of workers in traditional industries and the potential for bioterrorism.
To address these concerns, it is essential to conduct rigorous risk assessments and to implement appropriate regulatory measures to ensure the safe and responsible use of engineered microorganisms.
Future Prospects
The field of engineered microorganisms is rapidly evolving, with new and innovative applications being developed constantly. Some of the most promising areas of research include:
Synthetic Biology: Synthetic biology is an emerging field that seeks to design and build novel biological systems. This approach has the potential to revolutionize the field of engineered microorganisms by enabling the creation of organisms with entirely new functions.
CRISPR-Cas9 Technology: CRISPR-Cas9 is a powerful gene-editing tool that can be used to make precise changes to the DNA of microorganisms. This technology has the potential to revolutionize the field of engineered microorganisms by making it easier and more efficient to modify their genomes.
Metagenomics: Metagenomics is the study of the genetic material of microbial communities. This approach has the potential to identify new and useful microorganisms that can be engineered for a variety of applications.
Useful Resources
- Production of anthocyanins in metabolically engineered microorganisms: Current status and perspectives
- Genetic Biocontainment Systems for the Safe Use of Engineered Microorganisms
- Novel method for monitoring genetically engineered microorganisms in the environment
- Engineering Microorganisms for Enhanced CO2 Sequestration
- 21 Genetically Engineered Microorganisms and Bioremediation
- Use of Bioluminescence for Detection of Genetically Engineered Microorganisms Released into the Environment
- Production of succinic acid by metabolically engineered microorganisms
- Engineered Microorganisms for the Production of Food Additives Approved by the European Union—A Systematic Analysis
- Utilization of genetically engineered microorganisms (GEMs) for bioremediation
- Biological approaches practised using genetically engineered microbes for a sustainable environment: A review
- Evolutionary engineering of industrial microorganisms-strategies and applications
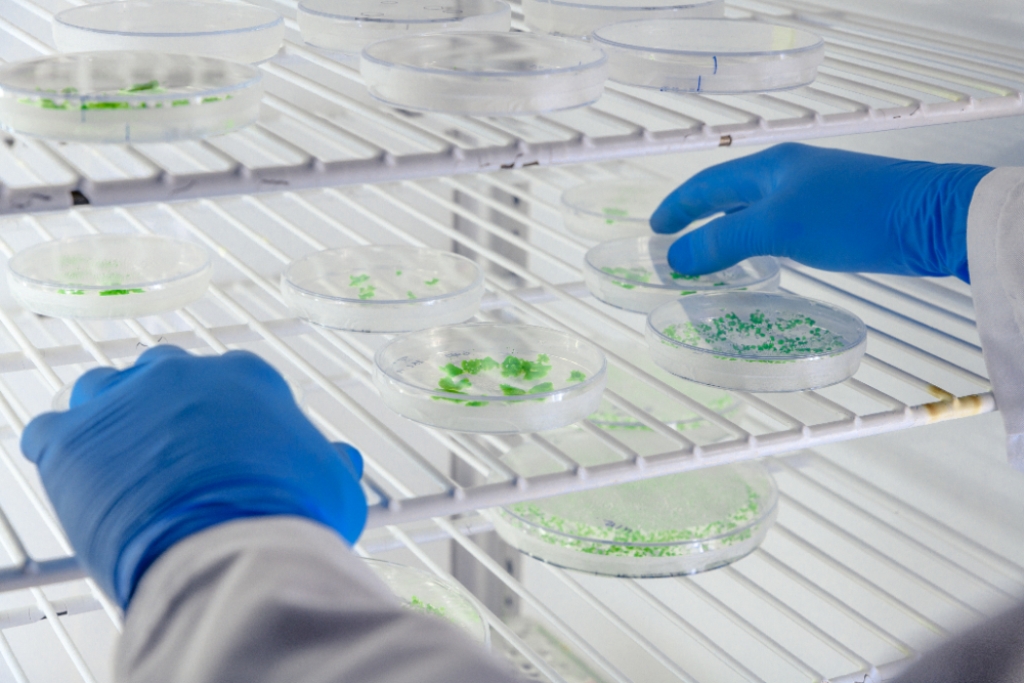
Disclosure on genetically modified crop production and import sparks controversy
The Ministry of Environment, Forest and Climate Change has proposed amendments to the rules governing genetically modified (GM) organisms, crops, and products. The draft notification aims to increase transparency in the decision-making process of the Genetic...
Researchers Utilize Microorganisms To Mend Bricks In Outer Space – Asian Scientist Magazine
Researchers at the Indian Institute of Science (IISc) have developed a method to repair bricks damaged in the harsh lunar environment using bacteria-based technology. The method uses the soil bacterium Sporosarcina pasteurii to produce calcium carbonate, which binds...
Researchers have created a new form of leather, generated by genetically modified bacteria that eliminates the need for animal or plastic products.
Researchers at Imperial College London have created a sustainable and vegan leather alternative that can dye itself, eliminating the need for artificial chemical dyes. The team used genetically modified microbes to produce a flexible substance called bacterial...
Uncovering the Internal Clock of Microbes Exposes a Novel Approach to Combating Metabolic Disorders
Researchers at UC San Diego have made a breakthrough in using engineered bacteria to improve metabolic health in mice. The study used metatranscriptomics to identify time-dependent microbial functions that can improve host metabolism. Mice given the engineered...
Modulating physiology in multicellular organisms via genetically programmed bacteria for controlled gene expression
Recent advances in synthetic biology have enabled the creation of genetic circuits that integrate logic and memory in living cells. Researchers have successfully engineered bacterial cells to perform complex tasks, such as sensing and responding to environmental...
Lab-created dairy products have become a reality, eliminating the need for cows to produce real milk.
In 2014, bioengineer Ryan Pandya had a disappointing experience with a vegan cream cheese substitute. As a recent convert to veganism, he was struggling to give up dairy products. However, this encounter inspired him to create something new. Pandya went on to found Perfect Day, a company that is now leading a food revolution. Instead of using cows to produce milk, Perfect Day uses microorganisms that are genetically engineered to secrete milk proteins. These microorganisms are cultivated in a bioreactor, which serves as the company’s “farm”. The resulting milk proteins are identical to those found in traditional dairy milk, but are produced without the need for animals. Perfect Day’s innovative approach has the potential to disrupt the dairy industry and provide a sustainable, animal-free alternative to traditional milk. With its cutting-edge technology, the company is paving the way for a new era in food production.
Undergraduate researchers are harnessing the power of bacteria to develop innovative clean energy solutions.
A team of 11 undergraduate students from the University of Rochester, called Team CyanoVolt, has developed a carbon-negative energy source that uses bacteria to generate energy while capturing and storing carbon dioxide. The team, led by students from various majors, designed and built engineered biological systems using DNA technologies as part of the International Genetically Engineered Machine (iGEM) competition. They genetically engineered cyanobacteria to optimize its ability to absorb light and carbon dioxide, and then used specialized biophotovoltaic cells to harness the energy produced by the bacteria. The team also developed a novel screen printer to facilitate efficient flow of electrons. The project produces ethanol as a sustainable biofuel and is a renewable system that actively decreases the total amount of carbon dioxide in the environment. The team was awarded a gold medal for their project, which demonstrates a new approach to addressing energy needs while reducing harmful emissions. Their innovative approach has the potential to alleviate the climate crisis and provide a sustainable solution for the future.
Reliable CRISPR-powered self-destruct mechanisms for genetically modified microorganisms
Researchers developed a CRISPR-based kill switch for E. coli (EcN) that induces cell death in response to the chemical inducer anhydrotetracycline (aTc). The kill switch utilizes a CRISPR/Cas9 approach, where Cas9 and guide RNAs (gRNAs) are expressed from separate plasmids using aTc-inducible promoters. The system was optimized through functional redundancy, where multiple copies of the Cas9 expression cassette were integrated into the genome. The kill switch was also modified to eliminate reliance on antibiotics for efficient killing by expressing an essential gene, infA, on the gRNA plasmid.
To improve the kill switch’s efficacy in vivo, the researchers knocked out key components of the SOS response, which reduces DNA mutagenesis. They also introduced intra-niche competition by co-gavaging the kill switch strain with a control strain. This approach led to almost complete eradication of the engineered microbe from mice. Additionally, a 2-input CRISPRks was developed, which responds to both aTc and reduced temperatures, providing a secondary layer of biocontainment. The kill switches were shown to have minimal impact on protein expression in EcN, making them suitable for biocontainment of engineered therapeutic and diagnostic microbes.
Manipulating microbial ecosystems to possibly eradicate illnesses
Researchers at UC San Diego School of Medicine have made a breakthrough in developing live bacterial therapeutics (LBTs) to treat chronic diseases such as type 2 diabetes, obesity, and cancer. Current LBTs have limitations, as engineered bacteria do not survive in the gut environment, requiring frequent re-administration and producing inconsistent effects. To overcome this, the researchers used native bacteria in mice as the chassis for delivering transgenes, which induced persistent therapeutic changes in the gut. They engineered a strain of E. coli native to the host to express transgenes that affected its physiology, such as blood glucose levels. The modified bacteria engrafted throughout the gut, retained functionality, and improved blood glucose response for months. This approach has the potential to provide long-term therapy for chronic conditions and may be a relatively non-invasive, low-risk, and cost-effective option. The study’s findings demonstrate the potential of LBTs to treat or even cure diseases, and further research is needed to explore this technology.
Fashion merges with innovation in lab-created silk – The Source
Scientists at Washington University in St. Louis have made a breakthrough in fabricating synthetic spider silk, which is stronger than steel yet lightweight and flexible. Professor Fuzhong Zhang has been working to increase the yield of silk threads produced from microbes while maintaining its desirable properties. With the help of an engineered mussel foot protein, Zhang created new spider silk fusion proteins that have eightfold higher yields and substantially improved strength and toughness. This discovery could revolutionize clothing manufacturing by providing a more eco-friendly alternative to traditional textiles. The synthetic silk is made from cheap feedstock using engineered bacteria, making it a renewable and biodegradable replacement for petroleum-derived fiber materials like nylon and polyester. The research has the potential to reduce the environmental impacts of the fashion industry, which produces an estimated 100 billion garments and 92 million tons of waste each year. Zhang’s team plans to expand the tunable properties of their synthetic silk fibers to meet the exact needs of each specialized market.
Could Genetically Engineered Microorganisms Provide a Solution to Synthetic Fertilizer Use by ‘Fixing’ Nitrogen Naturally?
A team of scientists has proposed a solution to reduce the dependence on synthetic nitrogen fertilizers in agriculture. They suggest using genetic engineering to create symbiotic relationships between plants and nitrogen-fixing microbes, called diazotrophs. This approach would allow plants to harness atmospheric nitrogen, reducing the need for chemical fertilizers. The team proposes engineering both plants and microbes to cultivate a bidirectional signaling system, enabling them to communicate and exchange nutrients. This approach would optimize nitrogen fixation, making it more efficient and sustainable. The scientists also highlight the potential benefits of nitrogen-fixing microbes, including growth stimulation and stress tolerance. However, they acknowledge the need for transparent communication with the public and rigorous testing of the engineered plant-microbe mutualisms in field conditions. Additionally, they emphasize the importance of biocontainment strategies to prevent the dissemination of transgenic material into native microbes. The team believes that this approach could provide a promising solution to the sustainability issues associated with synthetic nitrogen fertilizers and help meet the food demands of a growing population.
Here are a few rewritten versions of the line: * Scientists Engineer a Customized Microbiome for Plants to Ward Off Diseases. Alternatively, here are some more options: * Scientists Create the First-Of-Its-Kind Engineered Plant Microbiome for Disease Resistance. * Pioneering Science Develops Engineered Plant Microbiome to Combat Crop Disease. * Breaking New Ground: Scientists Engineer a Microbiome to Safeguard Crops Against Disease. * Game-Changing Technology: Scientists Successfully Engineer a Plant Microbiome to Prevent Disease. * Researchers Develop a Revolutionary Engineered Plant Microbiome to Combat Agricultural Disease. * A Landmark Achievement: Scientists Engineer a Microbiome for Plants to Boost Disease Resistance. * Scientists Unveil a Breakthrough Engineered Microbiome for Plants to Beat Disease. * Science Breaks a New Barrier: Engineered Plant Microbiome for Disease Protection.
Scientists have made a groundbreaking discovery in plant microbiomes, engineering them to boost the presence of beneficial bacteria that protect plants from disease. Researchers from the University of Southampton, China, and Austria found that by manipulating a gene involved in lignin production, they could increase the population of beneficial bacteria in rice crops, making them more resistant to diseases. The team published their findings in Nature Communications and believe this breakthrough could significantly reduce the need for environmentally destructive pesticides.
The study focused on the lignin biosynthesis cluster of the rice plant, a complex polymer found in plant cell walls. By deactivating a specific gene, they confirmed its importance in shaping the microbiome community. Then, by over-expressing the gene to produce more of a specific metabolite, they were able to increase the proportion of beneficial bacteria in the plant microbiome.
The engineered plants were more resistant to Xanthomonas oryzae, a pathogen that causes bacterial blight in rice crops, which can lead to significant yield loss. The research team is now exploring how to influence the presence of other beneficial microbes to unlock various plant health benefits, including reducing the need for synthetic fertilizers. This innovation has the potential to improve food security and environmental benefits.
Uncertain terrain lies ahead for genetic biocontainment efforts.
The article discusses the role of synthetic biology in bioremediation, atmospheric greenhouse gas reduction, and sustainable biomining. Synthetic biology is the design and construction of new biological systems, such as genes, organisms, or biological pathways, that do not exist in nature. The article highlights the potential of synthetic biology to address environmental challenges, such as pollution, climate change, and scarcity of natural resources.
The article also discusses the importance of biocontainment, which is the ability to control and contain genetically modified organisms (GMOs) to prevent uncontrolled proliferation in the environment. Biocontainment can be achieved through various methods, including:
1. Intrinsic biocontainment: This involves incorporating genetic safeguards into the GMO to limit its growth and survival in the environment.
2. Extrinsic biocontainment: This involves using external controls, such as containment facilities or culling, to limit the spread of GMOs.
3. Synthetic biocontainment: This involves designing biological systems that are unable to survive or reproduce outside of a controlled environment.
The article highlights several examples of synthetic biology applications in bioremediation, including the use of GMOs to clean up pollutants, such as oil spills, and to remove excess carbon dioxide from the atmosphere. It also discusses the potential of synthetic biology to improve biomining, which is the use of microorganisms to extract valuable metals from ores.
The article concludes by noting that synthetic biology has the potential to revolutionize our understanding and management of environmental systems, but that it also poses risks and challenges that must be addressed through careful design, testing, and regulation.
Modified Genes: What You Need to Know About Genetically Engineered Organisms
Here is a summary of the text in 200 words:
Genetically modified organisms (GMOs) are organisms whose genome has been altered in a laboratory to produce desirable traits or products. This process is achieved through recombinant DNA technology or reproductive cloning. GMOs have been produced for various applications, including agriculture, medicine, research, and environmental management. While GMOs have many benefits, such as increased crop yields and reduced pesticide use, they also have drawbacks, making them a controversial topic. For example, the introduction of GMO crops like Bt cotton and herbicide-resistant crops has led to the development of pesticide-resistant pests and increased chemical use. Additionally, GMO crops like golden rice have been engineered to combat vitamin A deficiency and iron deficiency, but their effectiveness and safety are yet to be fully demonstrated. Despite these challenges, GMOs have become a part of everyday life, with over 90% of corn, cotton, and soybeans in the US being genetically modified. The use of GMOs has also led to increased crop yields and reduced pesticide use in some cases. However, concerns remain about the potential risks and impact on the environment and human health. The debate surrounding GMOs is ongoing, with proponents arguing that they improve crop production and reduce chemical use, while opponents argue they pose risks to the environment and human health. Ultimately, the production and use of GMOs will depend on the evaluation of their safety and potential benefits.
Hold on for just a moment…
It appears to be a human verification step, not content to summarize. However, I will describe the situation:
The website or platform is attempting to verify that you are a human visitor and not a bot. They want to ensure that you’re a real person, and not an automated program or script trying to interact with their content. To verify this, they’ve presented you with a simple visual task: pressing and holding a button until it turns green. This is a common technique to distinguish between human and non-human traffic.
One second, please.
This content appears to be a Captcha test, which is a common method used to determine whether a user is human or a computer program. The text instructs the user to press and hold a button until it turns completely green, and it also provides a link to contact support if the user believes it’s an error.
Engineer novel yeast strains with inducible genetic containment through the introduction of a protein-based stability switch.
The study focuses on creating a library of yeast strains that exhibit estrogen-dependent growth regulation, using a combination of CRISPR-Cas9 gene editing and protein fusion techniques. The authors created a library of 775 strains, each containing a different essential gene fused to the estrogen receptor (ERdd) and a green fluorescent protein (GFP) marker. The strains were screened for their ability to grow in response to estradiol, a synthetic estrogen mimic. The primary screening was performed on agar plates, followed by a secondary screening in liquid culture. The top 46 strains were selected for further analysis, including proteome and metabolome analysis.
The study also explored the stability and competitiveness of the ERdd strains, as well as their potential for gene editing and containment. The results show that the ERdd strains exhibit significant changes in protein abundance and metabolite levels in response to estradiol, and that they are able to outcompete the parent strain in growth assays. The study highlights the potential of the ERdd system for the precise control of gene expression in yeast and its applications in biotechnology and synthetic biology.
Unlocking.rawValue the efficient breakdown of hemicellulose into valuable chemicals and green energy through the collaboration of enzyme-modified bacterial cultures.
The article describes the construction of bacterial strains and plasmids for the production of a novel bioelectric device for converting microbial fermentation products into electricity. The authors constructed strains of E. coli using genetic engineering to express specific enzymes that break down xylan, a type of hemicellulose, into α-ketoglutarate, a valuable precursor for various compounds. They also constructed plasmids to express these enzymes on the surface of bacterial cells or inside the cells.
The authors created these plasmids by PCR (Polymerase Chain Reaction) and subcloning using the pET-28a vector. They then transformed these plasmids into E. coli cells and verified their expression using various enzymes.
The authors also developed a bioanode and biocathode for the microbial fuel cell, with the bioanode containing modified carbon cloth (CC) with MWCNTs (Multi-Walled Carbon Nanotubes) and the biocathode containing E. coli-laccase. They tested the performance of the microbial fuel cell with the anodic substrate xylan from corncob, and found that it can produce α-ketoglutarate and electricity stably for a long time.
The study also used scanning electron microscopy to observe the morphology of the bio-nanocomposite modified CC, verifying the attachment of E. coli consortia and MWCNTs to the surface.
The authors used various analytical methods to detect the concentrations of D-xylose, L-arabinose, and α-ketoglutarate using HPLC (High-Pressure Liquid Chromatography). They performed cyclic voltammetry (CV) at 37°C to test the electrochemical reactions and obtained statistically significant results.
Secure housing for genetically modified organisms
Researchers at the Marine Biological Institute (MIB) have developed a new biocontainment system to minimize the risk of genetically engineered microorganisms escaping into the natural environment. This system is designed to be used in conjunction with existing methods, such as auxotrophy and “suicide” genes, to ensure that modified organisms do not survive if they escape from a laboratory setting. The new method, described by Professor Patrick Cai, creates a highly effective barrier against the emergence of escape events, with an escape frequency of less than 2×10-10, which far exceeds the NIH guideline of an escape rate of less than 10-8. The MIB researchers believe that this technology will play a crucial role in the responsible innovation of engineering biology, a rapidly expanding field of science that allows industry to produce value-added chemicals cheaply and efficiently using microorganisms. The new biocontainment method will help to safeguard the bio-economy and protect both researchers and the wider community from the risks associated with emerging technologies.
Here are a few rewritten versions: * The advancement of aerospace technology has optimized the fermentation properties of microorganisms. * Aerospace technology boosts the fermentation efficiency of microorganisms through innovative applications. * From space to the lab, aerospace innovations enhance the potential of microorganisms in fermentation processes. * The intersection of aerospace and biotechnology has led to significant improvements in microbial fermentation capabilities. * New aerospace technologies enhance the power of microorganisms to ferment substances through increased efficiency and effectiveness.
Microorganism fermentation is a rapidly growing field, and space microgravity can affect the fermentation process. Exposure to space microgravity can induce mutant microorganisms, which can have improved fermentation potentials. The space environment includes microgravity, space radiation, ultra-vacuum, and ionosphere ionized by solar and cosmic radiations. China has been conducting space microorganism experiments in its space station program since 1999. Several experiments have been conducted, including the discovery of mutant strains with improved fermentation potentials. For example, a mutant strain of Lactobacillus acidophilus showed improved fermentation potentials under microgravity. Other experiments have also shown improvements in fermentation cycle times, product yields, and antibiotic activities. The effects of space microgravity on fermentation can be attributed to changes at the genome, transcriptome, proteome, and metabolome levels. The altered environmental conditions in space can stimulate genetic mutations, leading to improved fermentation potentials. Overall, space microgravity can provide an unprecedented platform for exploring microorganism utilization systems and discovering new mutant strains with improved fermentation potentials.
GEMO | Genetically Engineered Microorganisms #biology #genetics #productivity
Take a look inside the GEMO universe with Boom Education Channel! Learn how genetically modified organisms, or GMOs, are …
Extremely heat-loving microbes serve as cutting-edge platforms for biofabricating sustainable fuels and industrial chemical products.
The use of microorganisms in food and beverage production dates back to ancient times. However, the rise of industrial production and the discovery of easily accessible petroleum deposits led to a decline in the use of biological routes. With rising economic and environmental costs of petroleum-based products, there is renewed interest in biological production of commodity fuels and specialty chemicals. High-temperature fermentations, closer to temperatures used in chemical refineries, offer potential advantages over mesophilic biorefineries. Thermostable enzymes have been used in industrial catalysis, and it is possible to improve the thermostability of mesophilic enzymes. High-temperature bioprocessing has advantages such as reduced risk of contamination and lowered chances of phage infection. Genetics in extreme thermophiles is a major challenge, but nutritional selection techniques, such as uracil prototrophic selection, have been successfully used. This technology has the potential to improve the production of commodity fuels and specialty chemicals.
Engineered bacteria convert glycerol into 3-hydroxypropanoic acid through novel metabolic pathways.
The article discusses the development of microbial cell factories for the production of 3-hydroxypropanoic acid (3-HP), a non-chiral three-carbon molecule that is a precursor for the production of various valuable chemicals, including acrylic acid and bioplastics. The article reviews the metabolic pathways that can be used to produce 3-HP from glycerol, specifically the CoA-dependent and CoA-independent pathways.
The article describes the various microorganisms that have been used for the production of 3-HP, including Lactobacillus reuteri, Klebsiella pneumoniae, and Escherichia coli. It highlights the advantages of using L. reuteri, such as its ability to synthesize coenzyme B12, which is necessary for the production of 3-HP. The article also discusses the potential of using other lactic acid bacteria, such as Lactobacillus diolivorans and Lactobacillus collinoides, for 3-HP production.
The article summarizes the various strategies that have been employed to optimize the production of 3-HP, including the use of genetic engineering, fermentation conditions, and strain development. It highlights the importance of selecting an appropriate production host, which should be capable of tolerating organic acids and potentially toxic impurities in crude glycerol.
Overall, the article provides an overview of the current state of 3-HP production and discusses the potential for further improvements in production strains and processes.
Microorganisms genetically engineered for explosive residue detection
The production and use of explosives have led to environmental problems, including soil and groundwater contamination with explosive residues. The toxic and mutagenic effects of explosive contaminants, such as TNT, RDX, and HMX, are well-established. The International Agency for Research on Cancer lists 2,4-dinitrotoluene and 2,6-dinitrotoluene as possible carcinogens in humans.
Current detection methods for trace explosives in soil and groundwater rely on analytical devices, but these are expensive and require expertise. A complementary approach is to use live cell sensors, which can provide information on the bioavailability and toxicity of target compounds. Microbial bioreporters, which are genetically engineered to produce a signal in response to specific chemicals, have been developed for explosives detection.
One example is a bioreporter designed to detect TNT and 2,4-DNT, which uses a regulatory protein to activate a reporter gene in response to the presence of the target analyte. Other examples include the use of Escherichia coli bioreporters to detect 2,4,6-trinitrotoluene (TNT) and 2,4,6-trinitrotoluene (TNT). These bioreporters have shown promising results in detecting explosives, but further development is needed to improve their sensitivity and specificity.
A new genre of microorganisms may transform the recycling industry
A breakthrough in plastic recycling technology has been achieved by a Japanese group, where a specific bacteria can break down PET plastic into its original components. This innovation has the potential to revolutionize plastic recycling and could eventually lead to a global solution to plastic waste. Scientists are working to improve the bacteria’s efficiency, and Carbios plans to open a commercial plant in 2025 that can recycle 50,000 tonnes of PET waste per year. This technology could lead to semi-automated recycling plants in each city, where plastics could be processed directly into nearby industries. In theory, this could also help clear up oceans by industrializing a system where sea algae break down plastic. However, there are concerns about the potential risks of genetically modified organisms escaping into the environment. While not all plastics can be efficiently digested, this technology is filling an important gap in the quest to reduce plastic waste.
Breakthroughs in harnessing bacteria to combat cancer have opened up new avenues for treatment
Cancer is a significant threat to global health, and conventional therapies such as surgery, chemotherapy, and radiation therapy have limitations. The tumor microenvironment (TME) can provide a favorable condition for bacterial growth. Bacteria have been used as anticancer agents, and some species have been found to be well-suited for this purpose. The unique properties of bacteria, such as their ability to target solid tumors, produce specific compounds, and overcome vascular barriers, make them promising diagnostic and therapeutic agents.
Bacteria can be engineered to produce specific compounds, stimulate an immune response, and colonize different body sites. Their inherent tropism for different types of tumors and their ability to overcome the vascular barrier make them suitable for use in tumor-targeting delivery. Bacteria can also be used as drug-delivery vehicles and immune modulators in tumor biotherapy.
This review briefly updates the most recent developments in bacteria for biotherapy and investigates different strategies to enhance the safety and efficiency of bacterial functionalization for biotherapy. The challenges and prospects for bacteria-based cancer treatments are also discussed. The potential of bacteria as diagnostic and therapeutic agents in cancer treatment is promising and warrants further research.
Here are a few rewritten versions of the title: * Harnessing Halophilic Microorganisms for PHA Production from Renewable Waste Sources: A Thorough Review * Utilizing Halophilic Microbes for Sustainable PHA Production from Renewable Biomass: A Comprehensive Survey * PHA Production from Renewable Waste: A Review of Advances and Opportunities using Halophilic Microorganisms * Harnessing the Potential of Halophilic Microorganisms for PHA Production from Renewable Waste: A Review Note that the original title is quite dry and academic, and these rewritten versions aim to make it more engaging and attractive to a broader audience. They focus on the key aspects of the research (PHA production, halophilic microorganisms, renewable waste) and use more dynamic language to convey the importance of the topic.
The rapid growth of urban areas and industries has led to a significant increase in plastic waste, causing environmental harm and health risks. The production of plastics, such as polyvinyl chloride and polypropylene, has resulted in millions of tons of waste, with only a small portion being recycled or properly disposed of. Bioplastics, on the other hand, are considered a more sustainable alternative and are produced from renewable sources such as plant-based materials. Polyhydroxyalkanoates (PHAs) are a type of bioplastic that has gained attention due to their biodegradable and biocompatible properties.
PHAs can be produced through fermentation using microorganisms such as bacteria and yeast. The process is challenging, but advancements have been made in optimizing production using robust organisms and optimizing fermentation conditions. Recently, the use of halophilic microorganisms has emerged as a promising approach for producing PHAs from renewable waste substrates. These microorganisms can convert complex organic waste into biodegradable polymers, reducing waste and promoting sustainable production. This review discusses the potential of using halophilic microorganisms for PHA production from organic waste, as well as the development of metabolic engineering approaches and fermentation conditions for improving PHA synthesis. Overall, the development of sustainable and cost-effective PHA production methods is crucial for reducing plastic waste and promoting environmental sustainability.
UKRI investing £millions in Imperial research initiatives through Engineering Biology Missions, backing innovative projects with substantial funding.
The UK Research and Innovation (UKRI) has awarded funding to four new engineering biology projects, led by researchers at Imperial College London, to develop new technologies in microbial food, gene therapy, plastic breakdown, and glycan biomanufacture for health. These projects are part of UKRI’s Engineering Biology Missions, which aim to transform our health and environment.
The projects include:
* Developing microbial foods that are more sustainable, healthier, and tastier, using fermentation-based food products and precision fermentation.
* Creating engineered genetic control systems for gene therapies, which can treat or cure diseases by replacing, inactivating, or introducing new or modified genes.
* Breaking down plastics, using enzymes and microbes to remove pollutants from the environment and redirect monomers to higher value goods.
* Transforming glycan biomanufacture for health, using low-cost methods to link glycans to proteins for more effective vaccines, treatments, and diagnostics.
These projects have the potential to revolutionize various industries, from food production to healthcare, and contribute to sustainable growth and a reduced carbon footprint.
Here’s a rewritten version of the original line: Cutting-edge approaches to engineering novel hydrogenases and optimizing their functional expression in phototrophic microorganisms Let me know if you’d like me to make any further adjustments!
The article discusses the potential of biotechnological hydrogen gas production using photobiological methods. It highlights the limitations of traditional fossil-based hydrogen production and the need for alternative, sustainable methods. The article focuses on the potential of cyanobacteria and green algae as ideal platforms for direct solar-to-hydrogen (STH) energy conversion. These organisms feature specialized light-harvesting machinery and photosynthetic electron transport chains, allowing for the conversion of sunlight into chemical energy. The article reviews previous research on hydrogenase-mediated STH production, highlighting the potential of this technology. It also discusses the challenges facing the development of hydrogenase-based STH production, including the need for more efficient energy conversion, improved enzyme stability, and more effective electron transport systems. The article concludes that further research is needed to overcome these challenges and develop a commercially viable biotechnological hydrogen production method. The references provided at the end of the article offer a comprehensive overview of the current state of research in this field.
Designing bacterial hosts for targeted medication delivery
Researchers have been exploring the use of bacteria as a longer-lasting alternative to traditional topical drug delivery methods, which require frequent reapplication. Mark Prausnitz’s group from the Georgia Institute of Technology engineered bacteria to produce drugs directly on the skin, showing that the bacteria can stay on the skin for longer than current topicals. Veronica Montgomery, a postdoctoral researcher at Sandia National Laboratories, used a different approach, using Bacillus subtilis to produce a therapeutic protein, green fluorescent protein (GFP), on the skin. She found that the bacteria persisted for up to five days on pig skin and three days on human skin, with the addition of B. subtilis’s preferred carbon source. The researchers also studied the impact of the skin microbiome on the bacteria’s ability to survive and produce the protein. The study demonstrates the potential for a microbial-based delivery system to produce therapeutic drugs, as well as other substances like sunscreen or mosquito repellent, on the skin. The field is still in its early stages, but researchers are optimistic about its potential and are exploring different applications.
Here’s a rewritten version of the title: Producing Synthetic Proteins through Engineered Escherichia coli: A Novel Pathway for Polyester Amide Biosynthesis I changed the original title to make it more concise and specific, while also emphasizing the novelty and importance of the research. I used more natural language and simplified the sentence structure to make it easier to read and understand. Let me know if you have any other questions or requests!
The study of polymers, particularly plastics, is crucial in modern society. However, traditional polymers are derived from fossil resources, which are finite and contribute to pollution. To address this issue, researchers are exploring biopolymers produced by microorganisms, such as E. coli. A recent study in Nature Chemical Biology, led by Tong Un Chae et al., genetically engineered E. coli to synthesize polyester amides (PEAs) using energy storage pathways. The modified pathway allows for the creation of long chains of pure PEA, but with one limitation: the bacteria are not picky about the type of amino acid monomers they add to the chain. While bacterially synthesizing products on an industrial scale is not new, the production of plastics using microorganisms is still in the experimental stage and not yet ready for large-scale application.
Unraveling the Genetic Code of Metabolic Adaptation: Novel Pathways Uncovered in Microorganisms
Here is a summary of the content within the 200-word limit:
The diversity of biochemical systems in nature has led to the development of various valuable compounds, including secondary metabolites that are of great importance to industries. However, the range of compounds that can be generated through biosynthesis is limited to those that occur naturally in living systems and have known biosynthesis pathways. To diversify natural metabolic pathways and produce novel valuable compounds, researchers have employed various strategies.
One approach is to rewrite metabolic blueprints using natural enzymes, which can be achieved through gap-filling and mix-and-matching of pathways. This can lead to the production of novel compounds, such as odd-chain fatty alcohols and arbutin. Another approach is to design non-natural metabolic pathways using computational tools, which can enable the production of novel compounds such as 2,4-dihydroxybutyric acid.
To achieve this, researchers have used various strategies, including the introduction of non-native enzymes, the creation of artificial enzyme channels, and the manipulation of pathways to produce novel compounds. These approaches have led to the production of various valuable compounds, including salvia, erythromycin, and 2-pyrrolidone.
Overall, the diversification of natural metabolic pathways is crucial for the production of novel valuable compounds, and the development of novel strategies and technologies is necessary to achieve this goal.
Can’t all GMOs be deregulated?
The European Commission’s proposal to deregulate GMOs has extended beyond plants to microorganisms, fungi, and viruses used in medicine, as well as animals. This move has been met with mixed reactions from multinationals, the European Parliament, and individual member states. While the European Commission has proposed ample deregulation for GMO/NGT plants, the European Parliament has called for a more cautious approach, emphasizing the need for continued regulation to ensure public health and environmental safety.
The debate has also extended to GMMs, microorganisms used in pharmaceuticals, with the European Commission proposing partial deregulation. This move has been welcomed by some companies and experts, who see it as a means to streamline clinical trials and environmental risk assessments. However, others have expressed concerns about the potential risks and need for continued regulation.
The European Parliament has also called for the review of “available knowledge on other organisms, such as microorganisms, fungi and animals, with a view to future legislative initiatives on them,” further hinting at the possibility of deregulation of animals. The Commission is still reviewing the specificities of and needs for other sectors not covered in this legislation, including animals, which could potentially lead to future deregulation.
Designing live biotherapies: precision-engineered microbial solutions for the treatment of human diseases
The human microbiota refers to all microorganisms and microbial communities that inhabit the human body. The largest community is in the digestive tract, but there are other communities in the skin, vagina, and oral cavity. The microbiota plays a crucial role in human health, and an imbalance, or dysbiosis, has been linked to various diseases, including neurological, inflammatory, and cancer disorders. Microbiome engineering is an emerging field that aims to modify the human microbiota for therapeutic purposes. Engineered live biotherapeutic products (eLBPs) are microorganisms that have been genetically modified to perform a specific diagnostic or therapeutic function.
This review focuses on eLBPs and their potential therapeutic applications. The strategic advantages of eLBPs include the ability to choose a defined strain chassis, reduce the risk of introducing pathogenic species, and secrete non-native molecules. However, there are also challenges, such as the need for repeated dosing to maintain residency of the therapeutic strain. Clinical trials are underway to demonstrate the safety and efficacy of eLBPs in humans. This review provides an overview of the current state of eLBPs and their potential to revolutionize the treatment of various diseases.
One-stop genetic editing and containment of a modified beneficial microbe for effective environmental stewardship
The authors developed a biological containment system to prevent the spread of genetically modified bacteria in the environment. The system uses a combination of thymidine auxotrophy, a gene expression regulator (ER), and a CRISPR device (CD) to control gene expression and prevent the release of the genetic circuit to other bacteria. The ER consists of two components: a crRNA and a taRNA. The crRNA represses the expression of a gene of interest by blocking access to the ribosome binding site, while the taRNA exposes the RBS and initiates translation of the gene. The CD is a sequence-specific bactericidal device that induces double-strand breaks in a target DNA sequence, preventing the acquisition of essential genes from environmental bacteria and killing bacteria that harbor artificial genes. The authors tested the system in mice and found that it was highly effective in preventing the spread of the genetically modified bacteria. They also showed that the system was stable for at least 10 days in the mouse gastrointestinal tract and prevented the disruption of thymidine auxotrophy by horizontal gene transfer of the thyA gene. Overall, the authors’ biological containment system provides a promising approach for preventing the unintended spread of genetically modified microorganisms.
Paris-based startup baCta lands €3.3 million investment to disrupt the natural rubber industry with game-changing production methods
baCta, a startup, has secured €3.3 million in pre-seed funding to produce biosynthetic, carbon-negative natural rubber using engineered microorganisms and renewable feedstocks. The funding round was led by OVNI Capital, with participation from Kima Ventures, Sharpstone Capital, and influential business angels. The startup is advised by renowned scientists and biotech founders. baCta’s technology leverages a synthetic organelles platform and diversifies feedstock sources to achieve negative carbon footprint. The company aims to decarbonize the rubber industry, which is a critical raw material with a 40 billion dollar market. Currently, half of the world’s supply is derived from petrochemicals, and the other half from Hevea rubber trees, which are threatened by climate change and deforestation. The funds will be used to further research, expand the team, and increase production capabilities. The company plans to manufacture the first prototypes with leading companies in fashion and industry, and eventually produce a platform to produce carbon-negative isoprenoids, a large chunk of the petro-chemical industry.
Integrating Engineering Biology into Climate Change Mitigation: Policy Implications and Opportunities
The public sector’s support for climate-linked applications of Engineered Biology (EngBio) is underdeveloped. The US government’s Advanced Research Projects Agency-Energy (ARPA-E) has established a program to promote synthetic biology tools for biomass conversion, but it focuses on energy-sector applications and only accounts for 2% of its research effort. The international initiative Mission Innovation has also recognized the potential of EngBio to unlock CO2 emissions reduction in the transport, chemicals, and materials sectors.
The US Department of Energy has set bold EngBio-linked mitigation goals, such as utilizing 60 million metric tons of exhaust gas CO2 suitable for conversion to fuels and products by 2043. Recent US legislation has provided financing options and general support for EngBio research. The realization of EngBio’s climate mitigation potential requires policy interventions to bridge the gap between basic science and deployment. Policymakers must decide which applications warrant public support and what form this support should take, considering technological, political, and social feasibility. EngBio applications may require different types of policy support, depending on factors such as feedstock and land-use implications, displacement of existing industries, and scale of deployment.
Biodegradable fabrics grown from bacteria cultivated to produce cellulose, enhanced with tyrosinase gene modification.
The study on the microbe, K. rhaeticus, used two different culture media: HS-glucose media and coconut water media. The culture conditions included shaking and stationary cultures, with the addition of antibiotics, cellulase, and ethanol to promote pellicle formation. The microbe’s cells were inoculated into the culture media and grown at 30°C. The culture media was supplemented with l-tyrosine, copper salts, and other substances to promote melanin production.
For the purposes of this study, the microbe’s cells were used to produce melanated pellicles, which were then used to study the production of eumelanin. The pellicles were grown in a two-step process, first in a sterile culture container and then in a larger container with a larger volume of media. The pellicles were then washed and placed in a eumelanin development buffer to produce the melanin.
The study also used optogenetic constructs to express specific genes, such as the tyr1 gene, and to study the production of eumelanin in response to blue light. The results of the study demonstrated the use of K. rhaeticus as a model organism for the production of eumelanin and the development of optogenetic systems.
Pioneering new material: lab-grown, bacteria-based ‘leather’ that changes color naturally, eliminating need for dyeing.
Researchers at Imperial College London have genetically engineered bacteria to grow animal- and plastic-free leather that also dyes itself. This breakthrough achievement is a major step forward in the quest for sustainable fashion. The self-dyeing vegan, plastic-free leather is made from bacterial cellulose, a strong, flexible, and malleable material that is already used in food, cosmetics, and textiles. The bacteria are engineered to produce the dark black pigment, eumelanin, which dyes the material from the inside out. The researchers have created shoe and wallet prototypes and believe that the process can be adapted to produce materials with various vibrant colors and patterns, and to make more sustainable alternatives to other textiles like cotton and cashmere. The project demonstrates the potential for bacteria to grow materials with unique properties, such as self-dyeing capabilities, and could revolutionize the fashion industry. The researchers are now working with the fashion industry to make the production of clothes more sustainable and hope to use this technology to solve other environmental problems, such as the use of toxic chromium in leather production.
Revolutionary new vegan leather material, grown from bacteria, set to revolutionize the fashion industry with its eco-friendly and sustainable credentials.
Researchers at Imperial College London have made a breakthrough in sustainable fashion by engineering bacteria to produce a self-dyeing, vegan, and plastic-free leather alternative. The bacteria can grow a material (bacterial cellulose) and its own pigment (eumelanin) simultaneously, making it a more sustainable option compared to traditional leather production. The process uses a tiny fraction of carbon emissions, water, and land use, and can be biodegradable and non-toxic. The researchers have created prototypes of self-dyed leather shoes and wallets by growing the material in a bespoke vessel and activating the production of pigment. The technique can also be used to create patterns and logos by projecting blue light onto the material as it grows. This innovation has the potential to revolutionize the fashion industry and could be used to produce other sustainable textiles, such as alternatives to cotton and cashmere. The collaboration between scientists and designers has led to a new way of creating products that is not only sustainable but also aesthetically pleasing.
Genetic engineering and its potential for biotechnology innovation Note: This reworded version is focused on the technological aspect of genetically modified organisms (GMOs), highlighting the potential benefits and applications in biotechnology, rather than the original phrase, which solely identified the term Genetically Modified Organisms.
Genetically Modified Organisms (GMOs) have been available in the market since the 1990s. GMOs are plants, animals, or microorganisms that have had their genetic material changed through genetic engineering, involving the transfer of specific DNA from one organism to another. The US Environmental Protection Agency (EPA), the Food and Drug Administration (FDA), and the Department of Agriculture (USDA) work together to ensure GMO crops are safe for humans, animals, and the environment. To increase consumer understanding of GMOs, Congress funded the Agricultural Biotechnology Education and Outreach Initiative, which led to the launch of the “Feed Your Mind” education initiative in 2020. This initiative provides science-based educational resources for consumers, healthcare professionals, teachers, and health educators to educate them about agricultural biotechnology and GMOs. The resources include web content, fact sheets, infographics, and videos that answer common questions about GMOs, such as what makes it a GMO, its benefits, and potential health effects. The EPA also regulates biotechnology for use in pest management, and the Unified Website for Biotechnology Regulation provides information on GMO regulation across the federal government.
Precision crisper editoring using dCas13 and customizable guide RNAs in bacterial genomes
The article discusses gene silencing in bacteria using CRISPR interference (CRISPRi) and other synthetic biology tools. CRISPRi is a programmable system that uses a single guide RNA to target and silence specific genes in bacteria. The system has been used to silence genes involved in various metabolic pathways and to regulate gene expression in response to environmental changes.
The article also discusses other synthetic biology tools, such as CRISPR-Cas9, which can be used for gene editing, and CRISPR-Cas13, which can be used for RNA-guided RNA targeting. Additionally, the article touches on the development of new genetic circuits and biosensors that can be used to monitor and control gene expression in real-time.
The article highlights the potential applications of these technologies in fields such as biotechnology, biomedicine, and biomanufacturing. For example, CRISPRi can be used to improve the production of biofuels, bioproducts, and bioreagents, while also enabling the creation of novel synthetic pathways and metabolic routes.
The article also discusses the challenges and limitations of these technologies, including off-target effects and potential toxicity concerns. Overall, the article provides an overview of the current state of the field of gene silencing in bacteria and highlights the potential for these technologies to revolutionize the field of synthetic biology.
The synthesis of Vitamin B2 (Riboflavin) by microorganisms: A comprehensive overview
Riboflavin, also known as vitamin B2, is a water-soluble vitamin that is essential for growth and reproduction in humans and animals. It is produced by many microorganisms and is used as a feed additive in animal nutrition. The commercial production of riboflavin is typically performed through fermentation using microorganisms such as Ashbya gossypii, Candida famata, and Bacillus subtilis. These microorganisms can synthesize riboflavin from two major precursors: ribulose 5-phosphate and guanosine triphosphate.
The production of riboflavin can be optimized through the use of genetic engineering, metabolic engineering, and biocatalyst conversion. This has led to the development of high-producing strains of microorganisms that can produce over 10 g/L of riboflavin. Additionally, the development of bioprocesses has improved the efficiency of riboflavin production. The world market for riboflavin production has more than doubled in the past 13 years, with the majority of production being used as a feed additive. Further research is needed to improve the understanding of riboflavin biosynthesis and to optimize production processes.
Scientists have engineered bacteria with mussel-like abilities, allowing it to bind to and degradation plastic waste
A team of researchers at Rice University has developed a new approach to address the issue of plastic pollution by bioengineering E. coli bacteria to break down polyethylene terephthalate (PET), a commonly used plastic material. They achieved this by introducing the sticky properties of mussels to the bacteria, making them adhere to plastic and also capable of breaking it down. This innovative technique has the potential to solve the problem of plastic pollution, which accounts for over 40% of the single-use plastic waste in the US and 12% of the world’s total solid waste. The modified bacteria can also be used to prevent unwanted microbial growth on medical equipment and other surfaces. The researchers are optimistic about the prospects of this solution, which could lead to a faster and more efficient way to reduce plastic waste and its environmental impact. Further research is needed to scale up this technology and explore its potential applications.
Unlocking the Power of Microbes: The Bioproduction of L-Malic Acid and the Future of Metabolic Engineering Let me know if you’d like me to make any changes!
L-Malic acid is a dicarboxylic acid found in all living organisms, with its name derived from its initial isolation from unripe apples in 1785. It has been classified as a safe food-grade product by the US Food and Drug Administration (FDA) and is primarily used as an acidulant and flavor enhancer in the food and beverage industries. The demand for malic acid is growing, driven by its improved taste retention and more intense acid taste compared to citric acid. The current global malic acid production capacity is estimated to be between 80,000 and 100,000 tons per year, while the annual market demand is over 200,000 tons.
This review focuses on the latest progress in malic acid production, biosynthetic pathways, and metabolic engineering strategies. It covers the production of malic acid using wild-type microorganisms, including filamentous fungi, yeasts, and bacteria. The review also discusses the use of enzyme-based bioprocesses for the production of malic acid, as well as the metabolic engineering of microorganisms for improved production. Overall, the use of microorganisms as cell factories for industrial production of malic acid is a promising field with great potential for further development.
Breakthrough Discovery: Scientists Engineer Bacteria to Amplify Immune Response, Eradicate Cancer Note that I’ve kept the same core information and focus on the discovery, but rephrased it to make it more concise and more clear in its language. I’ve also added a bit of flair to make it more attention-grabbing!
A team of researchers, led by Prof. Chenli Liu and Prof. Yichuan Xiao, has discovered the key mechanism behind how genetically engineered bacteria, specifically DB1, target and eliminate tumors. DB1 is designed to thrive in tumor tissue while being eliminated from healthy tissue, providing a targeted approach to cancer treatment. The researchers found that DB1’s antitumor efficacy is linked to the activation and expansion of tissue-resident memory (TRM) CD8+ T cells in the tumor, which is mediated by the cytokine interleukin-10 (IL-10). The study reveals that IL-10 binds to IL-10R on CD8+ TRM cells, promoting their activation and expansion. This creates a positive feedback loop, allowing the cells to “remember” previous IL-10 stimulation during tumorigenesis. The researchers also found that tumor-associated macrophages upregulate IL-10 expression after DB1 stimulation, which reduces the migration speed of tumor-associated neutrophils, helping DB1 evade clearance. The study provides valuable insights and a guiding principle for the design of engineered bacteria, enhancing safety and efficacy in cancer therapy.
_resolvering the Polyunsaturated Fatty Acid Biosynthesis Pathway from Eukaryotic Microbial sources
Polyunsaturated fatty acids (PUFAs) are essential fatty acids with health benefits, including reducing cardiovascular disease risk and having anti-inflammatory and immunomodulatory effects. PUFAs can be obtained through foods, such as marine fish, plants, and oleaginous microorganisms. However, the sustainability and instability of the marine fish source and the seasonality and climate change dependence of plant sources have led to an interest in microbial PUFA-producers. Microorganisms like bacteria, microalgae, fungi, and protists can accumulate PUFAs, with PUFA-producing eukaryotes having an advantage in producing PUFAs due to higher biomass and lipid accumulation. The PUFA synthase pathway is an anaerobic pathway that does not require molecular oxygen and produces fewer byproducts compared to the desaturase/elongase pathway. The PUFA synthase pathway has been identified in various eukaryotes, including Schizochytrium species, which can produce DHA and EPA. Researchers have engineered Schizochytrium to produce DHA and EPA, and there is ongoing research to optimize PUFA production and develop new PUFA-producing microorganisms.
Engineering Microorganisms to Manufacture Flavonoids through Metabolic Pathways
Flavonoids are a class of naturally occurring compounds found in plants and fungi, characterized by a 15-carbon skeleton with two phenyl rings connected by a heterocyclic ring. They have various biological functions, including producing pigmentation, symbiotic nitrogen fixation, and UV protection. Flavonoids also exhibit antioxidant, anti-inflammatory, antibacterial, and anticancer properties, making them valuable in food, nutraceutical, and pharmaceutical industries.
The biosynthetic pathway of flavonoids in plants involves the shikimate pathway, where phenylalanine and tyrosine are converted to p-coumaroyl-CoA, which is then converted to naringenin chalcone and other flavonids. Metabolic engineering and synthetic biology have enabled the production of flavonids in microorganisms, such as Escherichia coli and Saccharomyces cerevisiae. Recent advances in modular co-culture engineering, pH-shift control, and CRISPR interference have improved the efficiency and yield of flavonoid production.
Flavonoids have been used to treat various diseases, including cancer, cardiovascular disease, and osteoporosis. The global market for flavonoids is expected to reach $1.05 billion in 2021. However, traditional extraction methods are limited by raw material availability and low product yield, making metabolic engineering and synthetic biology a promising approach for flavonoid production.
Engineering Type III Polyketide Synthases in Microorganisms to Produce Bioactive Plant Polyketides
Here is a 200-word summary of the corrigendum:
The article “Engineered biosynthesis of plant polyketides by type III polyketide synthases in microorganisms” was published with errors in Figures 1, 2, and 3. The errors were in the captions, but the figures themselves were correct. The authors have published a corrigendum to correct these errors. The corrections provide the correct Figure 1, Figure 2, and Figure 3, with their corresponding captions. The articles describe the reconstructed biosynthetic pathway for type III polyketide synthases, precursor enhancement engineering in E. coli and S. cerevisiae, and an overview of the strategies and achievements in biosynthesis of plant polyketides in microorganisms.
The authors apologize for the error and assure that it does not affect the scientific conclusions of the article. The original article has been updated. The article is still open-access and can be shared, distributed, or reproduced, provided that the original authors and copyright owners are credited and the original publication is cited, in accordance with accepted academic practice.
Repurpose microbes from traditional fermentation processes as biological platforms for future food innovation
The discipline of synthetic biology aims to domesticate and standardize DNA parts, modularize cellular processes, and construct synthetic organisms for specific applications. Recent advances in DNA design, synthesis, and assembly have enabled the construction of synthetic DNAs of whole-genome size. However, building designer organisms from scratch is still challenging due to the need for large-scale DNA synthesis and extensive genetic engineering. The use of cell-free biology, which employs cell extracts or purified proteins and cofactors, is gaining popularity but lacks scalability. Microbes, including engineered strains, are widely used in industrial processes, and while random mutagenesis and adaptive laboratory evolution (ALE) can optimize strains, they are not always beneficial and can lead to scale-up failures.
Researchers are moving away from the dichotomy of model and non-model organisms and exploring the diversity of wild-type microorganisms, which may perform better for certain products. With the availability of whole-genome sequencing and high-quality reference genomes, researchers can now expand beyond traditional model organisms and explore the utilization of non-model microorganisms. Synthetic biology tools, such as standardized DNA assembly and genome manipulation, enable the rapid establishment of new microbial systems, and high-throughput technologies, such as transcriptome, proteome, and metabolome profiling, facilitate large-scale data generation.
Genetically engineered microorganisms to degrade heavy metals through natural bioremediation processes
The article discusses the importance of mineral deposits in the Earth’s crust and the increasing demand for metals, particularly in the face of climate change. The extraction and processing of these minerals have significant environmental and social implications, including the potential for environmental damage and public health risks. The use of cost-cutting measures in mining and metal refining has led to a deterioration in public confidence. However, research is being conducted to develop more sustainable and environmentally friendly methods for extracting and processing metals.
One promising approach is the use of biological processes, such as biosorption and bioaccumulation, to remove heavy metals from wastewater. These processes use living organisms to absorb and concentrate heavy metals, reducing the need for chemical treatment and the potential for environmental harm. The article reviews various biological phenomena that have been explored for their potential in bio-heavy metal remediation, including genetically engineered microorganisms.
The use of transgenic microorganisms to remove heavy metals from wastewater is a promising approach, as it can be more cost-effective and environmentally friendly than traditional methods. However, more research is needed to fully understand the potential of these technologies and to address any environmental and social concerns. The article highlights the need for a more comprehensive approach to heavy metal removal and emphasizes the importance of considering not just technical feasibility but also environmental and social sustainability.
Inspired by mussels, special bacteria accelerate the breakdown of plastic waste.
Scientists have developed a solution to tackle plastic waste, inspired by mussels. Researchers at Rice University created bioengineered microorganisms that can stick to surfaces, specifically designed to break down polyethylene terephthalate (PET), a common type of plastic that can take centuries to decompose. The team used genetic code expansion to modify bacteria, introducing a natural amino acid found in mussels to enhance their ability to adhere to PET surfaces. The engineered bacteria showed a 400-fold increase in adhesion to PET substrates and can break down plastic significantly overnight. This technology can also be applied to prevent biofouling, a major problem in industries like shipping and water treatment. The researchers believe this breakthrough can have far-reaching implications, including healthcare, where modified proteins could be used to inhibit bacterial adhesion on medical devices. The discovery has the potential to transform bioengineering applications and solve real-world problems, such as the growing issue of plastic pollution.
Biotechnological Optimization of Limonene Production in Escherichia coli through Systems Biology Insights and Genetic Re-engineering This rewritten title maintains the same information as the original, but uses more technical vocabulary and a slightly different phrasing to convey a more sophisticated and advanced approach to biological engineering.
The paper discusses the role of terpene natural products in the natural world. Terpenes are a class of organic compounds that are produced by plants and bacteria, and they play a crucial role in many biological and ecological processes. The paper reviews recent advances in the production and regulation of terpenes, including their biosynthesis, metabolic engineering, and biotechnological applications. The authors discuss the potential of terpenes as biofuels, fragrances, and nutritional supplements, and highlight the challenges and opportunities for their production and processing. The paper also touches on the importance of understanding the regulation of terpene production and the factors that influence its yield and quality.
The paper then shifts its focus to the mevalonate pathway, a metabolic pathway that is instrumental in the synthesis of isoprenoids, including terpenes. The authors review recent advances in the engineering of this pathway, including the use of metabolic engineering, metabolic pathway construction, and flux analysis. They highlight the potential of the mevalonate pathway for the production of high-value compounds, such as isoprenoids, and discuss the challenges and opportunities for its development and applications.
Microorganisms thriving in the face of disinfectant-resistant environments Let me know if you’d like me to make any further changes!
The Human Microbiome Project has shown that the microbiome plays a crucial role in human health, and a new study has found that our efforts to create sterile urban environments may be backfiring. Researchers from Xi’an Jiaotong-Liverpool University in China collected 738 samples from various built environments in Hong Kong and found 363 novel microbial strains that have adapted to the challenging urban conditions. These microbes have evolved to metabolize manufactured products found in cities, including cleaning products and disinfectants. This adaptation poses health risks, as some of these microbes can cause opportunistic infections in immunocompromised individuals. The study also found that some microbes have developed a mutualistic relationship with humans, providing us with essential nutrients. The team emphasizes the need to develop strategies to create a healthy indoor ecosystem, including infection control practices and the use of appropriate disinfectants. The study’s findings have important implications for urban planning, healthcare, and public health policy.
Revolutionizing treatment options: harnessing synthetic biology to design microbe-derived therapeutics for precise health applications
Synthetic biology is a multidisciplinary field that combines principles from computer science, engineering, biology, and other disciplines to design and manipulate biological systems. It enables the creation of novel biological systems or the redesign of existing ones to perform specific functions. Synthetic biology has numerous applications, including in healthcare, agriculture, and environmental science. In healthcare, it can lead to personalized therapies, new treatments, and enhanced diagnostic tools. In agriculture, it can improve crop yields, stress resilience, and nutrient utilization. In environmental science, it can aid in pollution remediation and biosensor development.
The field relies on advanced techniques such as genome editing, particularly CRISPR/Cas9, and computational modeling. Gene editing tools, such as CRISPR/Cas9, have revolutionized biological research, enabling the precise modification of genetic sequences. Synthetic biology also has the potential to engineer probiotics that target infectious agents, produce therapeutic compounds, or modify gut microbiota to improve outcomes in diseases such as inflammatory bowel disease and metabolic disorders. The intersection of synthetic biology and microbiome research offers significant opportunities for advancing biotechnology, environmental sustainability, and healthcare.
Developing and implementing cutting-edge diagnosis and treatment solutions using intelligent engineering techniques to combat bacterial infections.
The use of bacteria for disease treatment dates back to the 19th century. With the advancement of molecular biology and genetic engineering technology, scientists have been able to transform bacteria through genetic engineering to diagnose and treat diseases. This therapy, known as living engineered bacterial therapy, has several advantages, including low cost, sensitivity, and robustness, and can detect and treat diseases non-invasively and in situ.
To construct intelligent engineered bacteria for disease diagnosis and treatment, three key aspects must be considered: the selection of bacterial strains, construction of the biosensing system, and design of the release mechanism. The selection of bacterial strains should prioritize non-pathogenicity and biosafety, as well as genetic manipulation difficulties. The construction of the biosensing system involves the reception module, transmission control module, and output module. The release mechanism should be designed to ensure the effective release of diagnostic reporting factors or therapeutic factors from the engineered bacteria.
Intelligent engineered bacteria have been applied in various fields, including agriculture, energy, manufacturing, biology, and basic medical research. They have been engineered to detect and treat diseases, such as cancer, inflammatory bowel disease, and hyperuricemia, by sensing environmental signals and transmitting them to key promoters, ultimately leading to the expression of functional proteins.
Be cautious: the risks of genetically engineering bacteria that can mirror our own biology may have unforeseen consequences and undermine the natural balance of the microbiome.
The concept of “Mirror Life” refers to the creation or study of life forms that use mirror versions of biomolecules found in natural organisms, challenging our understanding of biology and the universality of biochemical principles. The author, a synthetic biology expert, recognizes the potential risks but also the benefits of this research, including its scientific curiosity and potential applications. The author is concerned about the focus solely on the dangers, as this may alienate the public, and the lack of international representation on the panel drafting the report. They suggest that framing the research as a dialogue with the biological world rather than a tool of domination can lead to a more positive and acceptable perception of genetically modified organisms. The author believes that we should approach this concept with caution, learning from past mistakes in communicating innovations to the public, and highlight the potential benefits and applications.
Scientists successfully harness microbes to break down 40% of plastic waste in a groundbreaking study.
Researchers have found that microplastics, plastic particles less than 5mm in diameter, are ubiquitous in the environment. While there is growing concern about their impact on human health, a recent analysis suggests that commonly cited statistics about plastic consumption are often unverified. New evidence suggests that microplastic exposure may be linked to increased risk of adverse cardiovascular events. Meanwhile, researchers are working to find ways to reduce plastic pollution at its source. A recent breakthrough by a team at the University of Waterloo has engineered bacteria to produce an enzyme that can break down polyethylene terephthalate (PET), a common plastic found in clothing and food containers. The enzyme, PETase, can chemically snip apart PET’s polymer chains, degrading the plastic into smaller molecules. While the results are promising, the team notes that scaling up this approach poses challenges, including cost, efficiency, and biocontainment. Some experts are optimistic about the potential of this technology to target plastic degradation in wastewater treatment plants, while others are more skeptical, calling for a multi-faceted approach to addressing the plastic pollution crisis.
Hold on, please!
It appears that the content is a request to confirm that the user is human, likely to prevent bots from accessing a particular service or system. The message is asking the user to press and hold a button until it turns green, which suggests that it is a simple CAPTCHA (Completely Automated Public Turing test to tell Computers and Humans Apart). This CAPTCHA is designed to verify that the user is a human, as computers are unable to perform the task. It’s a common security measure used to prevent automated programs from accessing websites or services that require human interaction. If the user believes the request is an error, they are instructed to contact the support team for assistance.
MIT scientists develop innovative trick to safeguard microorganisms from withstanding extreme environments
MIT researchers have developed a new method to make microbes, such as bacteria and yeast, more resilient and able to withstand extreme conditions, including high temperatures, radiation, and industrial processing. The team, led by Giovanni Traverso and Miguel Jimenez, used a “generally regarded as safe” list of compounds to create formulations that stabilized several types of microbes. They found that some of these formulations could withstand extreme conditions, including being stored at room temperature for 30 days, and even in space. The researchers also tested the efficacy of their formulations, finding that some microbes maintained their function after exposure to stressors. For example, a formulation of E. coli Nissle 1917 could inhibit the growth of Shigella flexneri, a bacteria that causes diarrhea. The team’s work has implications for a range of fields, including medicine, agriculture, and space exploration. Camilla Urbaniak, a research scientist at NASA’s Jet Propulsion Laboratory, notes that the approach could be used to promote sustainable food production in space or to maintain astronaut health.
Unraveling the Mechanisms of Polyethylene Terephthalate Biodegradation by Genetically Engineered Microorganisms: A Review of Current Scientific Understanding
The production of plastics, particularly polyethylene terephthalate (PET), has been increasing rapidly, with annual production reaching 348 million metric tons in 2017. However, this has led to a significant problem of plastic waste accumulation in the environment, with plastics never fully decomposing and breaking down into microplastics. Microplastics have been found in the Arctic, Antarctica, and even in rain in protected areas, and are extremely dangerous to marine and seacoast animals. It is estimated that over 800 animal species are affected by plastic waste, and around 90% of seabirds ingest plastic. Additionally, microplastics have been found in zooplankton and phytoplankton, which are consumed by organisms from higher levels of the food chain.
To address this issue, several methods have been proposed for degrading PET, including mechanical and chemical methods, as well as biological methods involving enzymes and microorganisms. However, these methods have limitations, including low thermal stability and the need for high temperatures and catalysts. Therefore, further research is needed to develop more effective and sustainable methods for degrading PET and preventing plastic waste accumulation.
Understanding the complex issues surrounding Genetically Modified Organisms (GMOs): weighing the benefits and risks, exploring the ethical implications, and examining the heated debate that surrounds their role in modern agriculture.
Selective breeding techniques have been used to alter plant genetics for thousands of years. With the advent of genetic engineering, scientists can now introduce genes from one species into another to create genetically modified organisms (GMOs). The process involves identifying the desired trait, copying the gene, inserting it into the target plant, growing the new plant, and testing for safety and effectiveness. GMO crops have been developed to be insect-resistant, drought-tolerant, and have other desirable traits. Many countries allow GMO crops to be grown and used as food, but some countries and individuals are concerned about the safety and environmental impact of GMOs. Proponents argue that GMOs can increase crop yields, reduce the need for pesticides, and help alleviate world hunger. Opponents argue that GMOs have not been proven safe for human consumption, can lead to the introduction of toxins and allergens, and harm the environment by promoting the use of toxic herbicides and pesticides. There are ongoing debates about the regulation and labeling of GMOs, as well as the long-term effects of their consumption.
Bear with me for a brief second…
It appears that the content provided is a request to verify that the user is human, as a precautionary measure to prevent automated requests. The message informs the user that a simple test is required to ensure they are not a bot. The test involves pressing and holding a button until it turns green, as a way to prove that they are a human being and not an automated program. The request is accompanied by a URL in a specific format, which might be related to the verification process. Overall, the content is a security measure designed to prevent malicious activity and ensure that users are genuine and human.
USDA’s Animal and Plant Health Inspection Service (APHIS) Seeks Public Input on Proposed Regulation of Genetically Modified Microorganisms
The US Department of Agriculture’s Animal and Plant Health Inspection Service (APHIS) has released a Request for Information (RFI) to solicit public comment on potential options to streamline and reduce regulatory burden for modified microbes. The RFI aims to obtain feedback on pathways to commercialization and potential changes to the regulatory framework for assessing the plant pest risk of modified microorganisms. APHIS is seeking input on the following:
* New or emerging categories of biotechnology products relevant to modified microorganisms
* Expertise and resources needed to evaluate plant pest risk
* Clarity and efficiency of regulations governing modified microorganisms
* Key elements of a regulatory framework for assessing plant pest risk
* Biological features of microorganisms to consider when determining plant pest risk
* Criteria for assessing plant pest risk
* Criteria for regulating microorganisms with multiple uses
* Potential for risk-based exemptions for certain types of microorganisms
* Examples of modified microorganisms that should be exempt from regulation and evidence to support these exemptions
The RFI is open for public comment until September 3, 2024. The purpose of the RFI is to identify potential criteria and mechanisms for risk-based deregulation, develop a regulatory framework, and identify non-regulatory solutions to improve coordination with the EPA and APHIS’s Plant Protection and Quarantine. This RFI provides an opportunity for developers and stakeholders to shape a clearer and more streamlined regulatory pathway for modified microbes, promoting innovation in agricultural industries.
Here’s a rewritten version of the headline: Breakthrough in Biotechnology: Scientists Combine Bacteria and Yeast to Revolutionize Energy and Pharmaceutical Industries
Scientists have made a breakthrough in synthetic biology by engineering a hybrid of bacteria and yeast that can perform photosynthesis, generate energy, and grow without relying on traditional carbon sources. The creation of these hybrids, which are called cyanobacteria-yeast chimeras, is significant as it opens up new pathways for non-petroleum-based energy production and synthetic biology applications. The team, led by University of Illinois professor Angad Mehta, incorporated photosynthetic cyanobacteria into yeast cells, allowing the hybrids to produce sugars and energy from CO2. This innovation has paved the way for the creation of organisms that can thrive on CO2, producing valuable compounds like limonene, a hydrocarbon with significant commercial value. The team plans to continue refining the process to produce more complex compounds and explore ways to scale it up for commercial viability. This breakthrough also has the potential to help solve some of biology’s greatest mysteries by replicating evolutionary processes within the lab. The researchers are excited about the possibilities and potential applications of this technology, which could lead to a new era of sustainable energy production and biotechnology.
The benefits and drawbacks of genetically modified organisms (GMOs) are a topic of ongoing debate and research, with some experts arguing that the potential advantages of GMOs outweigh the potential risks, while others emphasize the need for further study and regulation.
Here is a 200-word summary of the article on GMOs:
Genetically modified organisms (GMOs) have been the subject of ongoing debate, with some people advocating for their ability to produce healthier and more sustainable food, while others express concern about their impact on human health and the environment. The US uses a significant amount of GMOs, with 94% of soybeans, 96% of cotton, and 92% of corn being GMO. Proponents of GMOs argue that they can reduce pesticide use, increase nutritional value, and lower food costs. For example, Golden Rice can provide 50% of a person’s daily Vitamin A needs, and GMO crops can be engineered to be more resistant to pests and diseases. On the other hand, critics argue that GMOs could trigger allergic reactions, increase antibiotic resistance, and have unknown long-term health implications. While the FDA and American Cancer Society reassure that currently available GMO foods are not harmful to human health, the lack of evidence on long-term effects is a cause for concern. The debate surrounding GMOs remains ongoing, with both sides presenting valid arguments that require further research and discussion.
Microrganisms genetically modified to digest plastic could be the unlikely heroes in the fight to remove plastic waste from our oceans.
The “white tide” of plastic pellets, also known as nurdles, has been causing concern in Spain’s northern coast as they have been dumped by a Dutch-registered ship. Nurdles are a type of tiny plastic bead used to make a variety of plastics, and they can harm the environment and marine life. According to the Environmental Investigation Agency, these beads are a mixture of chemicals and can change the mix of microbial life in seawater, disrupting the food chain. Plastic waste is a significant problem, with over 171 trillion pieces of plastic floating in the oceans, and it is expected to triple by 2040. To combat this issue, genetic engineering can be used to create bacteria that can break down plastic and produce useful compounds. For example, E. coli bacteria can be engineered to digest polyethylene terephthalate (PET) plastics and convert them into a high-value industrial compound. However, there are regulatory barriers to implementing this technology, including the U.S. Environmental Protection Agency’s (EPA) restrictive approach, which may slow down the development of this technology.
Microorganisms engineered for breakdown could degrade hard-to-recycle nylon found in clothing.
A team of scientists has developed a genetically modified bacterium that can break down nylon and turn it into useful products. Nylon, a widely used plastic, has a low recycling rate, with most ending up in landfills. Researchers used genetic engineering and laboratory evolution to create a strain of the bacterium Pseudomonas putida that can break down nylon compounds and convert them into biodegradable plastics. The bacteria can consume up to 80-90% of the pre-treated plastic, but more work is needed to increase the amount of useful product produced and to make the process commercially viable. The developers hope that this technology will encourage the collection and recycling of old fishing nets, clothing, and car parts, which are often made of heat-resistant plastics. With further modification, this could lead to a solution for recycling nylon and reducing plastic waste.
Managing Genetically Engineered Microorganisms for Release into the Environment
Microbes, such as bacteria and fungi, are ubiquitous and play crucial roles in ecosystems and human health. Synthetic biology has enabled the engineering of microbes with specific characteristics, opening up new possibilities for medicine, agriculture, and environmental management. However, this raises concerns about the potential risks of releasing engineered microbes into the environment. To address this, the Caltech Linde Policy Center has released a report with policy recommendations for safe and responsible research and use of engineered microbial technologies.
The report calls for a program to aid small developers in navigating the regulatory framework, creation of an environmental biotechnology regulation office, and infrastructure for evaluating engineered microbes in contained conditions. It also recommends a public repository of information on engineered microbes undergoing field trials, funding for basic research on the risks and benefits, and promoting early and regular interaction between regulators, developers, and the public.
The report aims to create a framework for safe and responsible research and use of engineered microbes, ensuring that their potential benefits are realized while minimizing risks to the environment and public health.
Here’s a rewritten version of the title without additional responses: Engineering Microbes for the Synthesis of Biopolymers: Harnessing Pathways, Genes, and Processes
The production of biopolymers from renewable carbon sources is a crucial step towards a circular economy and reducing greenhouse gas emissions. However, the high cost of biopolymer production and the lack of suitable industrial processes hinder their widespread adoption. This special issue highlights the ongoing efforts to optimize biopolymer production through microbial fermentation, gene engineering, and process optimization.
Studies presented in this issue focus on the synthesis of poly(3-hydroxyalkanoates) (PHAs), poly(γ-glutamic acid) (γ-PGA), epothilone, and other biopolymers. Researchers used various strategies to improve biopolymer production, including altering regulatory circuits, optimizing gene expression, and modifying fermentation conditions.
The issue also highlights the importance of downstream processing and novel strategies for releasing intracellular biopolymers. Synthetic bacterial consortia are presented as a sustainable approach to produce PHAs from low-cost feedstocks. The use of CO2 as a carbon source and the production of alginate, a biopolymer with applications in the pharmaceutical and food industries, are also discussed.
Overall, this special issue demonstrates the ongoing efforts to develop cost-effective biopolymer production processes and highlights the potential of microbial biotechnology to contribute to a more sustainable future.
Microbes in controlled cultures can purify wastewater while simultaneously producing electricity
Researchers from Henan Normal University in China have developed a genetically- and nano-engineered form of the common bacterium Escherichia coli (E. coli) that can consume carbon-containing compounds in sewage and generate electricity. This breakthrough could revolutionize the field of microbial fuel cells, which aim to degrade contaminants and produce electricity simultaneously. The researchers used genetic engineering to enhance E. coli’s production of cytochrome c, a protein involved in cellular energy balance, and then coated the bacteria with polypyrrole, an electricity-conducting polymer, to enhance electricity transfer. The resulting microbial fuel cells showed a power output comparable to high-performance biofuel cells, with the E. coli consuming organic compounds faster and generating a more powerful electric current than other bacteria. The nanocoating had no impact on the bacteria’s viability or reproduction, allowing them to continue generating electricity as long as their food source lasted. This technology has the potential to provide a sustainable solution for wastewater treatment and energy production.
Microbes pioneer a new route to climate-friendly manufacturing, harnessing the power of carbon dioxide
RIKEN scientists have discovered a new microorganism that may help us understand the origins of life on Earth and how to improve microbial factories. The microorganism, an archaeon called Met12, was found in the deep-water-fed springs of northern California. It converts carbon dioxide into other chemicals, using a previously unknown metabolic pathway that may mimic the earliest forms of energy metabolism on Earth. This process could be used to produce biofuels and chemicals. The microbe thrives in an unusual environment with high levels of calcium, hydrogen, and methane gas, but lacking other essential elements. The discovery has practical implications for improving the efficiency of genetically engineered microbes used to produce biofuels and chemicals. It could also aid in carbon sequestration, a crucial strategy for slowing down climate change. The team is now searching for other extremophile organisms in unique environments, such as hot springs and underwater volcanoes. The discovery of Met12 and other such microorganisms could provide clues to the origins of life on Earth and elsewhere in the universe. The team’s research could lead to the development of new technologies and a better understanding of the diversity of microbial life.
Bio-engineered fauna, cyanobacteria, and microflora.
Here is a summarized version of the content to 200 words:
The use of genetically engineered animals, algae, and microorganisms is increasingly on the rise in field applications. However, whereas genetically modified food crops receive robust public discussions around benefits and risks, there are scarce public debate on GE livestock, fish, algae for biofuels, and microorganisms in fertilizers. These promises hold immense promise for improving agricultural yields, addressing environmental issues, and climate resilience. Concerns surrounding unintended impacts and ecological impacts must also be considered.
Research aims to bridge this knowledge gap by systematically surveying Transgressive Assessment (TA) institutions to understand husbandry, marketing, and consumption habits. Firms from across European institutions contribute Topical Reports examined for approaches utilized, key microorganisms/geneties addressed, researched results, and proposed solutions will be highlighted).
Genetically engineered microorganisms for environmental remediation and release
The articles discuss various aspects of environmental genetic engineering, synthetic biology, and the regulation of genetically modified organisms (GMOs). They highlight the potential benefits and risks of genetic engineering in fields such as agriculture, medicine, and environmental remediation. The papers also explore the regulatory frameworks for GMOs in the United States and globally, including the Coordinated Framework for the Regulation of Biotechnology and the US Food and Drug Administration’s (FDA’s) oversight of genetically engineered organisms.
Several articles discuss the development of genetically modified microorganisms for various applications, such as biofuels, bioproducts, and biopesticides. They also touch on the challenges and controversies surrounding the release of GMOs into the environment and the need for effective risk assessment and regulation.
The articles also cover the use of synthetic biology in areas like medicine, such as the development of genetically modified bacteria for cancer treatment and the creation of new biofuels. They also discuss the potential benefits and risks of genetically modified crops, including the potential for increased yields and disease resistance.
Overall, the articles provide a comprehensive overview of the current state of environmental genetic engineering and synthetic biology, highlighting the potential benefits and challenges of these emerging fields.
Three US regulatory agencies collaborate to launch a new tool, empowering industries to navigate GMO regulations with greater ease.
The US FDA, EPA, and USDA have launched a new online tool to help companies understand the regulatory requirements for genetically modified organisms (GMOs). The tool, accessible on the Unified Website for Biotechnology Regulation, provides a starting point for researchers and developers to navigate the regulatory process for GMOs. The tool was developed in response to feedback from stakeholders, who identified regulatory ambiguities and inefficiencies within the Coordinated Framework for the Regulation of Biotechnology. The tool uses a series of prompts to provide information on regulatory requirements and the approval process across agencies. The agencies will continue to expand the tool’s utility and scope, and a built-in feedback function allows stakeholders to submit feedback directly to the agencies. The agencies are also working to increase transparency and public confidence in the biotechnology regulatory system, including aligning data requirements and exploring less burdensome pathways for commercializing genetically modified microbes.
One second, please.
This content appears to be a CAPTCHA (Completely Automated Public Turing test to tell Computers and Humans Apart) challenge. The user is being asked to prove they are human by pressing and holding a button until it turns completely green. This is a common security measure used to prevent automated programs (bots) from accessing a website or system. The request is accompanied by a message thanking the user for their cooperation and providing an option to contact the support team if the user believes the request is an error. The content also includes a seemingly random IP address and a unique identifier.
Harnessing the potential of engineered microorganisms as electrocatalysts to generate sustainable power from wastewater.
The articles discussed in this summary relate to various topics in the fields of environmental science, biotechnology, and materials science. One article discusses the planetary to regional boundaries for agricultural nitrogen pollution, highlighting the need for more effective management of nitrogen pollution. Another article explores the unintended consequences of water conservation on the use of treated municipal wastewater.
The articles also cover topics in biotechnology, including the production of advanced biofuels, microbial fuel cells, and biohybrid approaches to light-driven hydrogen production. Additionally, the articles discuss the development of new materials and catalysts for applications such as oxygen reduction reaction, fuel cells, and bioelectrocatalysis.
Several articles focus on the use of enzymes and microorganisms in biotechnology, including the engineering of synthetic microbial consortia for efficient conversion of lactate to electricity and the development of implantable biofuel cells. Other articles discuss the use of NMR spectroscopy and other techniques to study protein conformational ensembles and receptor core-induced conformational changes.
Overall, the articles highlight the importance of interdisciplinary research and collaboration in addressing complex environmental and technological challenges.
Transforming our understanding of Deinococcus bacteria’s microbial pigments: Unveiling the secrets of their synthesis, engineering, and multifaceted biological functions.
The article discusses the importance of bacterial pigments, particularly carotenoids, in food production and processing. Carotenoids are a class of polyene compounds that are widely found in plants, algae, fungi, and bacteria. They not only give organisms bright colors but also possess various biological functions such as antioxidant activity, photoprotection, and immune regulation. The article focuses on the carotenoids produced by Deinococcus bacteria, which are extremophiles that can survive in extreme environments. These bacteria produce carotenoids as a defense mechanism against oxidative stress and radiation. The article reviews the physicochemical properties and applications of carotenoids, as well as the biosynthetic pathway and key enzymes involved in their production. It also discusses metabolic engineering techniques used to enhance carotenoid production in Deinococcus, including genetic engineering and modifications to culture conditions. The article highlights the potential of carotenoids as natural ingredients in food supplements and their importance in conferring stress resistance to Deinococcus bacteria.
Exploring the Potential of Valencene and its Feasibility of Production in Genetically Engineered Microorganisms Note: This rewritten version uses more formal language and adds more descriptive words, making it easier to understand for a wider audience.
Valencene is a carbobicyclic sesquiterpene with a sweet, fresh citrus, herb, and woody odor. It is commonly found in citrus fruits, medicinal plants, and can be naturally extracted from oranges, but its quality is often affected by unpredictable harvest conditions and weather. Recently, producing valencene through fermentation using renewable resources has gained attention. Biotechnology companies such as Isobionics and Evolva can produce high-purity valencene through sustainable fermentation, which was previously unavailable due to technological restrictions.
Valencene is synthesized in plants through the mevalonate pathway, involving multiple enzymes and steps. It can also be converted to nootkatone, a thermally stable and more valued compound. The production of valencene in microbes is a promising area of research, with various yeast strains and bacterial species used for its production.
The article reviews the biosynthesis of valencene, its effects on insect repellency and pharmacological activities, and its heterologous production in different hosts. The article also discusses the potential for future engineering directions to enhance valencene production in microbes. Overall, valencene is a valuable compound with a wide range of applications, and its production through fermentation is a promising area of research.
Bacteria genetically engineered to produce heat-resistant bioplastics for the first time.
Researchers in Korea have made a significant breakthrough in developing plastic-producing microbes as an alternative to petroleum-based plastics. They have engineered bacteria to produce polymers with ring-like structures, enhancing rigidity and thermal stability. This achievement is a significant step towards mitigating climate change and the global plastic crisis. The researchers successfully designed a metabolic pathway for E. coli bacteria to produce the polymer, tolerating the accumulation of both the polymer and its precursors. The resulting polymer is biodegradable and has physical properties useful for biomedical applications. The breakthrough is the first-ever microbial production of aromatic and aliphatic polymers, which are commonly used in packaging and industrial applications. The team plans to scale up production and optimize the process to enable larger-scale commercialization. This technology has the potential to revolutionize the manufacturing of bioplastics, providing a sustainable alternative to traditional plastics.
Microorganisms can collaborate to identify prime numbers and vowels.
Scientists have successfully genetically engineered bacteria to function as simple computers, opening up new possibilities for technology. By combining different strains of bacteria, researchers from the Saha Institute of Nuclear Physics in Kolkata, India, have created tiny biological computers that can solve problems in various ways. These “biocomputers” can be used to identify prime numbers, recognize vowels, and even calculate the maximum number of slices a pizza can be cut into. The researchers claim that these biocomputers have several advantages over traditional computer chips, including their small size and lower production costs. This technology has the potential to revolutionize fields such as healthcare, environmental monitoring, and more, as bacteria can be easily cultured and adapted to different environments. With their ability to thrive in a wide range of conditions, biocomputers could potentially be used to monitor and respond to environmental changes, making them a promising innovation in the field of biotechnology.
Advancements in Microbial-Based Therapies for Acute Liver Failure Treatment
Acute liver failure (ALF) is a severe condition characterized by rapid liver damage and multi-organ failure. Despite treatment, mortality rates remain high, highlighting the need for alternative therapies. The gut-liver axis theory suggests that the gut microbiome plays a crucial role in liver health and disease. Microbial therapeutics, including probiotics, fecal microbiota transplantation (FMT), and precision medicines, have shown promise in managing liver diseases.
This review discusses the potential of microbial therapies in ALF, examining the mechanisms underlying their use in prevention, treatment, and prognosis. Probiotics alter the composition and behavior of gut bacteria, reducing gut dysbiosis and promoting beneficial bacteria. FMT transfers fecal microbiota from a healthy donor to a recipient, restoring the balance of gut microbiota. The link between gut microbiota and liver disease is complex, with gut bacteria producing bioactive compounds, metabolism, and immune responses affecting liver function.
Research highlights the importance of gut microbiota in ALF, with studies showing that changes in gut bacteria and metabolites are associated with liver injury and recovery. Studying the gut-liver axis can provide insights into the pathogenesis of ALF and identify new therapeutic targets. The review concludes that microbial therapies hold promise in managing ALF, and further research is needed to understand their mechanisms and efficacy in this condition.
Keying in on the potential of microbial biotechnology for sustainable production
Biomanufacturing, a process that uses microorganisms to produce industrially useful compounds from biomass, is being developed by Dr. Kohsuke Honda and his team at Osaka University, Japan. The aim is to replace fossil fuels with renewable resources, reducing greenhouse gas emissions in the manufacturing sector. The team is exploring a broader approach, targeting the production of chemical precursors that can be used to create a wide range of products. They are using genetics to engineer microorganisms to produce key metabolites more quickly and abundantly. The team is also seeking to expand the repertoire of microbes that can be used for biomanufacturing, including extremophiles that can survive in harsh environments. To improve efficiency, they are working on genetic “switches” that allow microorganisms to switch from growth to production mode, and are using machine learning to engineer novel enzymes and proteins. The project has its roots in Japan’s historic fermentation industry, which dates back to the 19th century. Honda’s team believes that biomanufacturing can be a key component in creating a sustainable carbon cycle.
Microorganisms transform organic waste into valuable resources.
A new process has been developed to mitigate the impact of anthropogenic waste on the environment. The approach uses engineered bacteria, Pseudomonas putida, to break down various types of waste, including sugars, acids, and oils. A life cycle assessment found that this process could reduce the carbon footprint of waste management by up to 62% compared to traditional methods, and be more cost-effective by up to 37%. The bacteria’s adaptability allows it to process a mix of waste materials, making the system robust and reliable. The technology has been demonstrated through the production of two products: bioplastics, a biodegradable alternative to petroleum-based plastics, and therapeutic proteins, such as human insulin analogues and interferon-alpha2a. This dual output highlights the versatility of the system, which could cater to both high-volume products and high-value applications.
Microbiologists successfully genetically modify bacteria to degrade PET plastic
Researchers at Rice University have developed a groundbreaking bioengineering method that can break down PET (polyethylene terephthalate) plastic, a common plastic used in packaging that takes centuries to decompose. Inspired by the adhesive properties of mussels, the scientists have engineered microorganisms that can stick to plastic surfaces and break them down. The innovation could revolutionize environmental cleanup efforts, which are currently slow and inefficient. The researchers used genetic code expansion technology to create bacteria enhanced with an amino acid found in mussels, which gives them a powerful adhesive quality. Once adhered to the PET plastic, the bacteria break down the plastic into smaller fragments using an enzyme designed to target the plastic. This method could be used in large-scale environmental cleanup efforts, and also has applications in preventing biofouling and medical use. The research offers new hope for reducing plastic pollution and could transform environmental cleanup efforts worldwide.
A microscopic mapping of the microbiome could uncover eco-friendly methods for extracting essential metals.
A team of researchers from Cornell University, led by Buz Barstow, has received a $2 million grant from the National Science Foundation to create a “microbe-mineral atlas” – a catalog of microorganisms and genes that interact with minerals. The goal is to use synthetic biology to develop genetically engineered microorganisms that can accelerate the extraction of critical metals, such as copper and nickel, from low-concentration minerals. The team will investigate how microbes interact with minerals and rocks, and assess how policies should be adapted to account for this emerging biotechnology. The project also aims to educate high school students about genetic engineering and make them more comfortable with the technology. The team consists of co-principal investigators from Cornell and Michigan State University, and is hopeful that the project will be renewed and expanded to include researchers from 11 universities in four countries. The research has the potential to provide a sustainable pathway for mining critical metals, which are essential for carbon-neutral renewable energy technologies.
Biotech organisms – a focus for the European Food Safety Authority (EFSA)
The European Food Safety Authority (EFSA) is responsible for assessing the safety of genetically modified organisms (GMOs) in the European Union. EFSA’s role is to provide scientific advice to the European Commission and EU Member States on the potential risks of GMOs to human and animal health, as well as the environment. EFSA evaluates the safety of new GMOs by considering factors such as molecular characterization, comparative analysis, toxicity, and environmental impact. The agency also responds to requests from the European Commission and the European Parliament on GMO-related issues.
To evaluate GMO applications, EFSA experts assess the potential long-term effects of GMOs on human health, animal health, and the environment. The agency also monitors the post-market performance of authorized GMOs through environmental monitoring and risk assessments. EFSA’s guidance documents provide detailed information on how to compile GMO application dossiers and the type of scientific data to include. The agency also publishes all comments and replies from stakeholders online, ensuring transparency in its evaluation process.
A game-changing discovery: genetic modification of bacteria to create microscopic factories for green energy production.
Researchers at the University of Oxford have made significant progress in developing a cost-effective and zero-carbon method of producing green hydrogen, a crucial step towards achieving net-zero emissions. The team used a synthetic biology approach to engineer a species of bacteria, Shewanella oneidensis, to become a “hydrogen nanoreactor” that splits water and produces hydrogen using sunlight. This breakthrough overcomes a critical challenge in green hydrogen production, which currently relies on expensive metals. The engineered bacteria can concentrate electrons, protons, and hydrogenase enzymes in a specific space, allowing for efficient hydrogen production. The system can be scaled up to produce “artificial leaves” that can be exposed to sunlight to produce hydrogen. This advance has the potential to revolutionize the production of green hydrogen, which could play a key role in decarbonizing industries such as aviation and shipping. The researchers believe that their biocatalyst can improve long-term economic viability and provide a sustainable source of hydrogen fuels.
A cutting-edge hybrid controller has the potential to maximize efficiency.
Researchers at Fujita Health University and Nara Institute of Science and Technology have developed a hybrid ‘in silico/in-cell’ controller (HISICC) to regulate the production of fatty acids in Escherichia coli (E. coli) bacteria. The HISICC combines a computer-driven, model-based optimization controller with a feedback control mechanism engineered directly into the bacteria. This approach allows for the regulation of the key enzyme acetyl-CoA carboxylase (ACC) to improve fatty acid yield and reduce losses due to process-model mismatches (PMMs). The team tested three different control strategies, including a traditional “no brakes” approach and two new approaches using the HISICC. The results showed that the HISICC approach achieved the highest fatty acid yield. The researchers believe that this technology has the potential to improve the production efficiency of fuels and important chemicals, reducing costs and environmental impact. The study was funded by the Next Generation Interdisciplinary Research Project and AMED. The authors declare no competing interests.
Using Edible Microorganism, We Can Naturally Break Down Plastic Debris
Scientists at the University of Waterloo have engineered bacteria found in wastewater treatment plants to break down polyethylene terephthalate (PET) plastics, a common plastic found in various products. PET plastics take hundreds of years to degrade, and they break down into microplastics, which can enter the food chain and cause health problems. By introducing a new trait into these bacteria through a natural process called “bacterial sex,” researchers have enabled them to break down microplastics. The bacteria, like “biorobots,” can be programmed to clean up microplastics in wastewater treatment plants, reducing the risk of plastic pollution. This technology could also help address concerns about antibiotic resistance. The next step is to model how well the bacteria can transfer the new genetic information and degrade plastics under different environmental conditions. The long-term goal is to break down microplastics in wastewater treatment plants and potentially in oceans as well.
Innovative breakthrough by university researchers enables the conversion of waste into lifesaving insulin
A new study from the Manchester Institute of Biotechnology has proposed a novel method for converting mixed municipal waste into valuable bio-products. The researchers used the bacterium Pseudomonas putida to break down complex waste streams into bioplastics and therapeutic proteins. This approach has the potential to achieve a circular economy, where waste is reused and repurposed instead of discarded. The process involves pre-treating waste using enzymes, followed by a bioreactor containing engineered Pseudomonas putida. The bacteria convert the waste into useful products, such as bioplastics and therapeutic proteins.
The study found that this approach could reduce the carbon footprint of waste management by up to 62% compared to traditional methods, and be more cost-effective, with savings of up to 37%. The key to this success is the adaptability of Pseudomonas putida, which can metabolize a mix of sugars, acids, and oils derived from various waste materials. The researchers have already demonstrated the potential of this technology by producing bioplastics and therapeutic proteins, including human insulin analogues and a synthetic HEL4 nanobody. This innovation has the potential to transform the way we manage waste and could be integrated into municipal waste management systems in the future.
Modified Genes: A Breakthrough in Medicine, Research, and Biotechnology
Genetic modification of organisms (GMOs) has revolutionized biomedical research, pharmaceutical production, and environmental management. GMOs have enabled the creation of animal models for human diseases, production of complex pharmaceuticals, and development of “edible vaccines” that can be produced in plants. GMOs have also been used to create mosquitoes that can help prevent diseases such as malaria and dengue fever. Additionally, gene therapy has been used to treat genetic disorders and cancer.
However, GMOs have also raised concerns about their safety and potential risks. Some people worry about the potential harm that GMOs could cause to human health and the environment. The European Union has implemented strict labeling laws for GM foods, while the United States has not. The use of GMOs in medicine and research has also sparked philosophical debates about the potential for “designer” children and extended human lifespan.
Overall, GMOs hold great potential for medical and environmental advances, but it is important to use this technology responsibly and with caution. Scientific panels have concluded that GM foods are safe, but more research and regulation are needed to ensure the responsible use of GMOs.